KRAS Target Analysis Report Summary
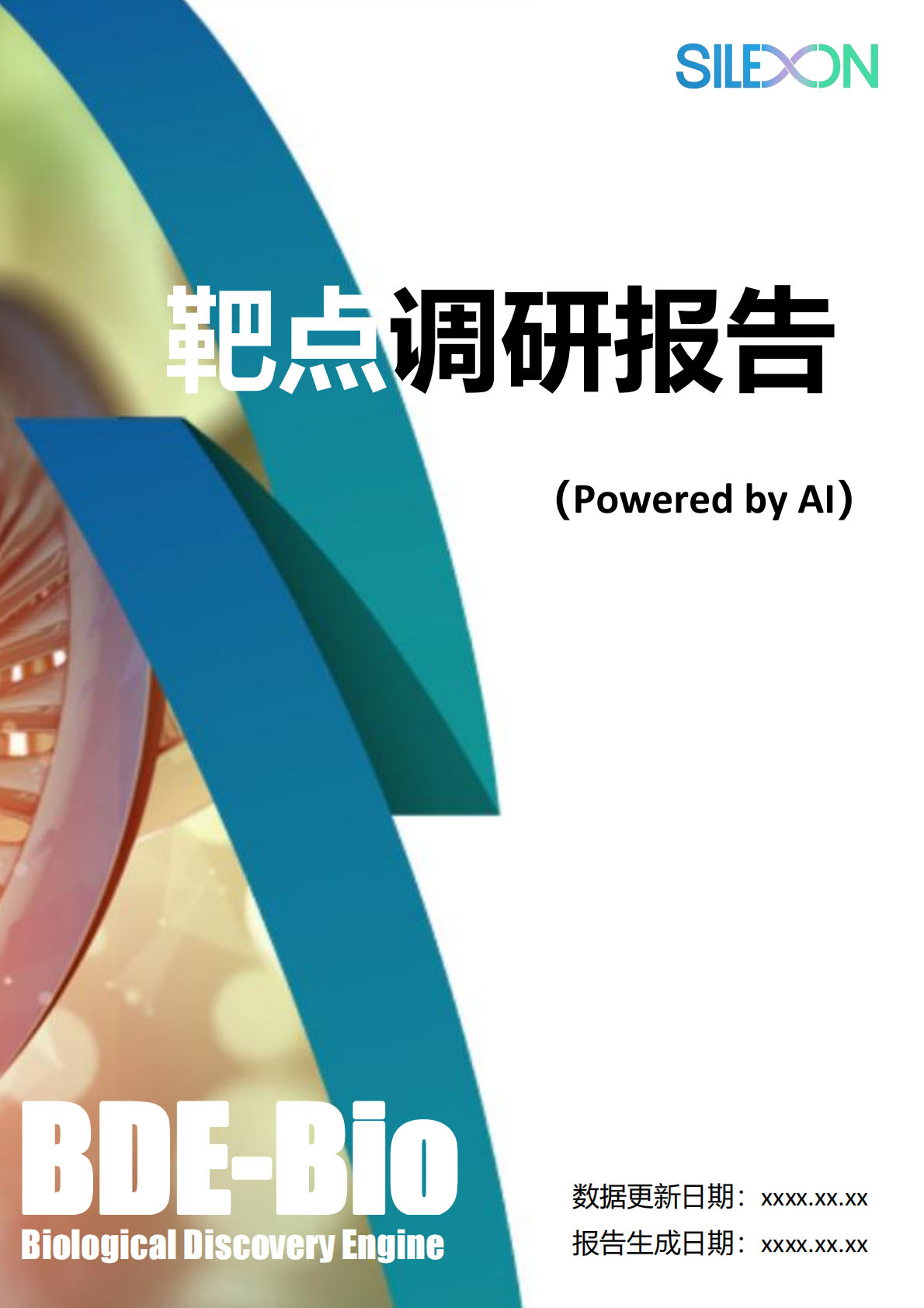

About the Target
KRAS, or K-ras, is a gene that plays a crucial role in cellular signaling pathways. It is involved in regulating the activation and deactivation of RAS proteins, which control cell growth and proliferation. Wild-type KRAS cycles between active and inactive states, while mutant KRAS is continuously active [1]. This activity is regulated by guanine nucleotide exchange factors (GEFs) that stimulate nucleotide exchange, and GTPase activating proteins (GAPs), which accelerate GTP hydrolysis [1][3].
In cancer, KRAS mutations are commonly found and can lead to constitutive activation of the protein [4]. These mutations, such as G12, G13, or Q61, prevent the normal regulation of KRAS and sustain its active state [1]. Consequently, this dysregulation of KRAS signaling can promote tumor growth and inhibit immune response [2]. In K-ras-driven models of non-small cell lung cancer (NSCLC), the presence of Th17 cells and IL17A in the tumor microenvironment enhances tumor cell growth and suppresses anti-tumor activity. This is due to the recruitment of myeloid-derived suppressor cells (MDSCs), increased regulatory T cells (Tregs), lactate production, and IL-6 expression [2]. In contrast, in Ptsd/d NSCLC models, Th17/IL17A inhibits tumor growth by promoting the recruitment of CD103+ dendritic cells to activate CD8 T cells for anti-tumor activity. This leads to the secretion of IFNgamma and the killing of tumor cells [2].
The structure of KRAS consists of effector lobes, allosteric lobes, a hypervariable region (HVR), and switch regions [1][3]. The G domains and HVRs are conserved among RAS isoforms, and RAS proteins cycle between GDP-bound inactive and GTP-bound active states [3]. GEFs stimulate the switch to RAS-GTP, while GAPs facilitate the termination of the active state [3]. The downstream effectors of active KRAS, such as RAF, PI3K, and RalGEFs, mediate cellular proliferation and differentiation signals [3].
Furthermore, KRAS activation involves GDP-GTP exchange mediated by GEFs, allowing for KRAS phosphorylation and subsequent effector functions [4]. This activation leads to the activation of multiple cellular proliferation pathways [4].
KRAS localization and interactions with other molecules in different cellular compartments also play a role in its functions. For example, calmodulin (CaM) can enhance K-Ras-driven stemness by inhibiting non-canonical Wnt signaling [5]. Other proteins such as PDE6D and NPM1 are involved in the dynamic localization and stabilization of K-Ras in different cellular structures [5].
In summary, KRAS is a key player in cellular signaling pathways that regulate cell growth and proliferation. Mutations in KRAS can lead to constitutive activation of the protein and contribute to tumor growth and immune response inhibition. The cycling between GDP-bound inactive and GTP-bound active states of RAS proteins is controlled by GEFs and GAPs. KRAS activation involves GDP-GTP exchange and is associated with multiple cellular proliferation pathways. KRAS localization and interactions with other molecules in various cellular structures also influence its functions.
Based on the provided context information, here is a comprehensive summary of the key viewpoints related to KRAS:
KRAS co-mutated subgroups have different immune phenotypes. KRAS/STK11 mutant tumors exhibit a poorly immune tumor micro-environment with low CD8 TILs and high T-regulator cells, while KRAS/TP53 mutant tumors show an immunogenic micro-environment with abundant CD8 TILs, activated dendritic cells, and fewer T-regulator cells [6].
KRAS4A, a palmitoylated GTPase, has a cycling mechanism between palmitoylated and depalmitoylated states. When palmitoylated, KRAS4A has a high affinity for the plasma membrane, but upon depalmitoylation, it gains affinity for endomembranes including the outer mitochondrial membrane (OMM). Tethering of KRAS4A to the OMM allows it to interact with HK1, enhancing HK1 activity and glycolytic flux [7].
Aspirin demonstrates potential in inhibiting the effect of EMT (epithelial-mesenchymal transition) in K-ras-expressing NSCLC cells. By inhibiting p65NFkappaB nuclear translocation, aspirin down-regulates Slug, a transcriptional repressor of E-cadherin, thereby reducing EMT and migration of NSCLC cells [8].
The K-Ras/MEK signaling pathway positively regulates Met mRNA levels, and this enhanced Met translation leads to increased cell proliferation. However, under certain culture conditions, K-Ras ablation down-regulates Met mRNA, resulting in the suppression of cell proliferation [9].
Activation of the RAS/ERK pathway, driven by EGFR activation, is believed to contribute to cellular proliferation in colorectal cancer. Patients with wild-type KRAS colon cancers benefit from anti-EGFR agents, while those with constitutively active KRAS mutants do not. However, the constitutively active KRAS G13D mutation is an exception, as patients with this mutation have shown a favorable response to anti-EGFR agents [10].
These viewpoints provide a comprehensive overview of the different aspects and roles of KRAS in various biological processes and cancer-related pathways.
Figure [1]

Figure [2]

Figure [3]

Figure [4]

Figure [5]

Figure [6]

Figure [7]

Figure [8]

Figure [9]

Figure [10]

Note: If you are interested in the full version of this target analysis report, or if you'd like to learn how our AI-powered BDE-Chem can design therapeutic molecules to interact with the KRAS target at a cost 90% lower than traditional approaches, please feel free to contact us at BD@silexon.ai.
More Common Targets
ABCB1 | ABCG2 | ACE2 | AHR | AKT1 | ALK | AR | ATM | BAX | BCL2 | BCL2L1 | BECN1 | BRAF | BRCA1 | CAMP | CASP3 | CASP9 | CCL5 | CCND1 | CD274 | CD4 | CD8A | CDH1 | CDKN1A | CDKN2A | CREB1 | CXCL8 | CXCR4 | DNMT1 | EGF | EGFR | EP300 | ERBB2 | EREG | ESR1 | EZH2 | FN1 | FOXO3 | HDAC9 | HGF | HMGB1 | HSP90AA1 | HSPA4 | HSPA5 | IDO1 | IFNA1 | IGF1 | IGF1R | IL17A | IL6 | INS | JUN | KRAS | MAPK1 | MAPK14 | MAPK3 | MAPK8 | MAPT | MCL1 | MDM2 | MET | MMP9 | MTOR | MYC | NFE2L2 | NLRP3 | NOTCH1 | PARP1 | PCNA | PDCD1 | PLK1 | PRKAA1 | PRKAA2 | PTEN | PTGS2 | PTK2 | RELA | SIRT1 | SLTM | SMAD4 | SOD1 | SQSTM1 | SRC | STAT1 | STAT3 | STAT5A | TAK1 | TERT | TLR4 | TNF | TP53 | TXN | VEGFA | YAP1