AKT1 Target Analysis Report Summary
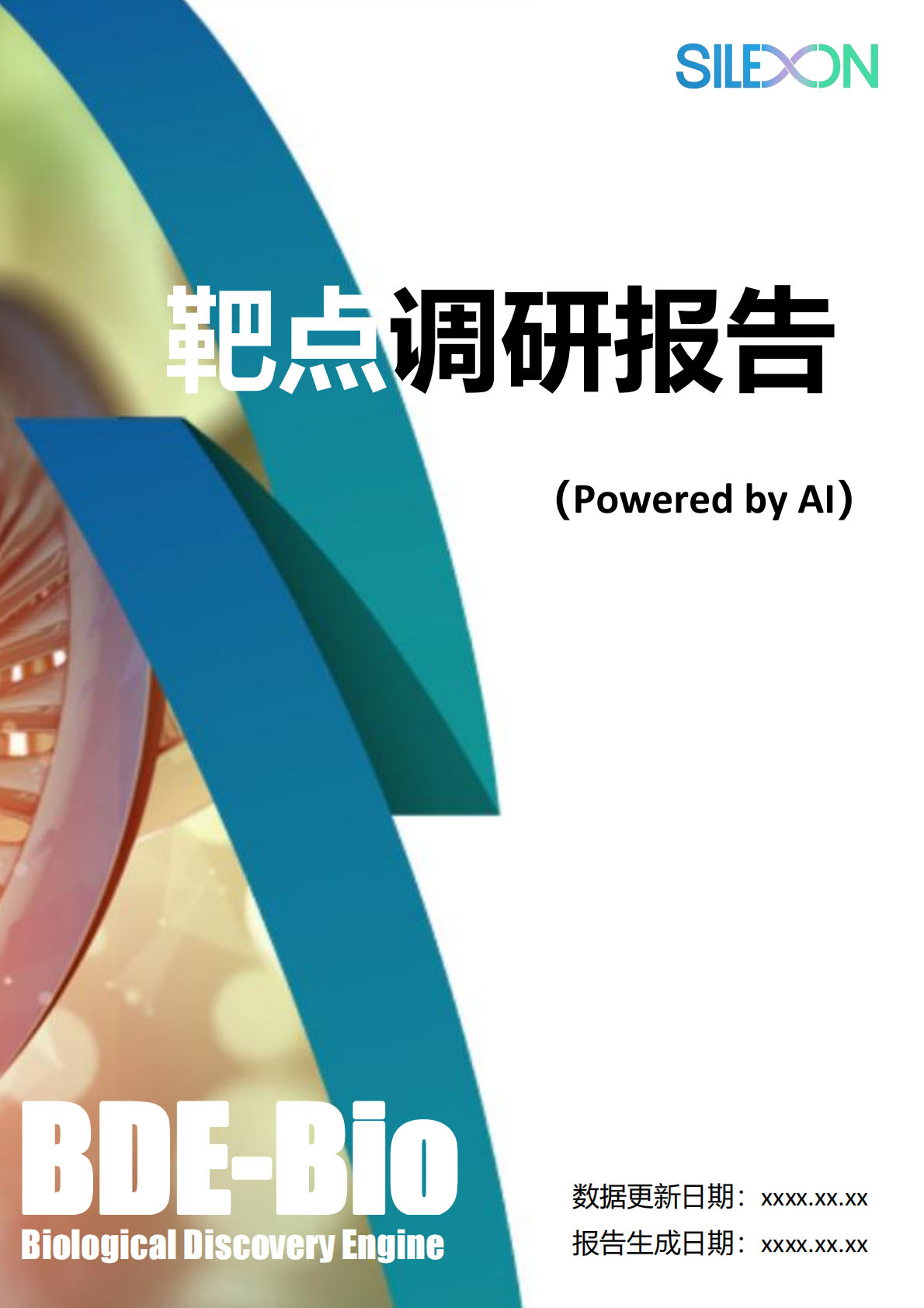

About the Target
AKT1, also known as Akt, is a key signaling molecule involved in various cellular processes and has been implicated in cancer progression and metastasis. The activation of AKT1 is crucial for its downstream functions, and dysregulation of AKT1 signaling contributes to the hyperactivation of the pathway in cancer [1].
There are different mechanisms that can lead to the hyperactivation of the Rac signaling pathway, which is interconnected with AKT1 signaling, in cancer. These mechanisms include reduced degradation via HACE1, altered Rac localization, mutations in Rho GDIs, and oxidation/reduction processes. These abnormalities further contribute to the activation of AKT1 [1].
In terms of AKT1 activation, the distribution of signaling phosphoinositides on endomembranes plays a critical role. The presence of PI(3,4,5)P3 and PI(3,4)P2 on specific membranes indicates potential sites of Akt activity. Additionally, various subcellular compartments contain bona fide Akt substrates that contribute to its biological functions [2].
Moreover, the activation of AKT1 in the presence of mutant BrafV600E and Cdkn2a loss plays a role in promoting lung and brain metastasis, and this effect is further enhanced by Pten loss. Pten is responsible for converting PIP3 to PIP2, thereby diminishing endogenous AKT1 activation. Conversely, loss of Pten leads to excess PIP3 and hyperactivation of AKT1, resulting in the emergence of lung metastases. Myristoylation of AKT1, which targets it to the plasma membrane, also leads to its activation and promotes metastasis [3].
Furthermore, AKT1 is involved in macropinocytosis, a process in which certain cup-like structures are formed in macrophages. AKT1 is activated upon the generation of PIP3 in these structures, and this activation is separate from macropinosome formation. PLCgamma and Akt contribute to the closure of the cup structure, which allows the macropinosome to separate from the plasma membrane and move towards the center of the cell [4].
Finally, the differentiation of human adipose-derived stem cells (hADSCs) is regulated by AKT1. KD025, an inhibitor, suppresses a pro-adipogenic regulator, leading to the inhibition of adipocyte differentiation in hADSCs. This mechanism overcomes the pro-adipogenic activity resulting from Akt activation and ROCK inhibition. In contrast, pan-inhibitors activate Akt and suppress ROCKs, promoting adipogenesis [5].
In summary, AKT1 plays a critical role in cancer progression and metastasis through its involvement in the Rac signaling pathway. Dysregulation of AKT1 activation can occur through various mechanisms, leading to the hyperactivation of the pathway. AKT1 is also involved in macropinocytosis and the differentiation of hADSCs. Overall, understanding the mechanisms of AKT1 activation and its downstream functions is crucial for developing targeted therapies against cancer and other diseases. [1][2][3][4][5].
From the given context information, several viewpoints can be extracted regarding AKT1:
AKT1 is involved in the activation of PI3K/AKT signaling pathways, which affects the expression of cell cycle and apoptosis-related proteins in AML-MDS cells, leading to cell cycle arrest and apoptosis [6].
Phosphorylation of Ser473 by mTORC2 activates AKT1 by relieving autoinhibition and promoting interactions with other proteins [7].
Insulin stimulation and oxidative stress can modulate AKT1 activation through redox-dependent mechanisms, such as recruitment to the plasma membrane or inhibitory disulphide formation, respectively [8].
AKT1 is part of a positive feedback network involving proteins integrin, Paxillin, Rac, PAK, and Merlin, which regulates focal adhesion and cell migration [9].
AKT1 plays a role in regulating TFEB nuclear translocation and activation. Inhibition of AKT activity promotes TFEB nuclear translocation, leading to enhanced transcriptional regulation of autophagy-lysosome pathways and increased cellular clearance [10].
Overall, AKT1 is a critical protein involved in various cellular processes, including cell cycle regulation, apoptosis, cell migration, and autophagy-lysosome pathways. Its activation and function can be influenced by phosphorylation, redox-dependent mechanisms, and interactions with other proteins.
Based on the provided context information, the PI3K/AKT/mTOR signaling pathway plays a crucial role in the communication between cancer-associated fibroblasts (CAFs) and cancer cells. The activation of this pathway in CAFs leads to their differentiation and influences various cancer behaviors, particularly cell proliferation [11][11].
The mechanism involves the activation of phosphatidylinositol-3-kinase (PI3K), which phosphorylates phosphatidylinositol-4,5-bisphosphate (PIP2) to phosphatidylinositol-3,4,5-trisphosphate (PIP3). miRNA-21 attenuates the inhibition of PTEN on PIP3, allowing PDK1/AKT signaling cascade to be initiated. This cascade ultimately transfers mTOR into the nuclei, regulating gene expression associated with CAF differentiation and motility [11].
Additionally, the Notch signaling pathway is involved in CAF differentiation through the AKT signaling pathway. B7-H3 promotes AKT phosphorylation, leading to proliferation in CAFs, whereas GW4064 inhibits migration through AKT phosphorylation [11]. Furthermore, CAF-derived factors such as HGF, IGF-2, IL-22, and CXCL5 activate the PI3K/AKT/mTOR axis, while CXCL12 inhibits PTEN. Activation of Smad2/3 by Nodal also induces AKT phosphorylation, and the collaboration of lncRNA UCA1 with mTOR further influences CAF-mediated PI3K/AKT signaling pathway, regulating cell proliferation, migration, and stemness in cancer cells [11].
Overall, the PI3K/AKT/mTOR signaling pathway is critical for the differentiation of various cells into CAFs and significantly impacts cancer behaviors, particularly cell proliferation. Moreover, the pathway is interconnected with other signaling pathways such as Notch and Nodal, and various factors secreted by CAFs further modulate this signaling axis [11].
Figure [1]

Figure [2]

Figure [3]

Figure [4]

Figure [5]

Figure [6]

Figure [7]

Figure [8]

Figure [9]

Figure [10]

Figure [11]

Note: If you are interested in the full version of this target analysis report, or if you'd like to learn how our AI-powered BDE-Chem can design therapeutic molecules to interact with the AKT1 target at a cost 90% lower than traditional approaches, please feel free to contact us at BD@silexon.ai.
More Common Targets
ABCB1 | ABCG2 | ACE2 | AHR | AKT1 | ALK | AR | ATM | BAX | BCL2 | BCL2L1 | BECN1 | BRAF | BRCA1 | CAMP | CASP3 | CASP9 | CCL5 | CCND1 | CD274 | CD4 | CD8A | CDH1 | CDKN1A | CDKN2A | CREB1 | CXCL8 | CXCR4 | DNMT1 | EGF | EGFR | EP300 | ERBB2 | EREG | ESR1 | EZH2 | FN1 | FOXO3 | HDAC9 | HGF | HMGB1 | HSP90AA1 | HSPA4 | HSPA5 | IDO1 | IFNA1 | IGF1 | IGF1R | IL17A | IL6 | INS | JUN | KRAS | MAPK1 | MAPK14 | MAPK3 | MAPK8 | MAPT | MCL1 | MDM2 | MET | MMP9 | MTOR | MYC | NFE2L2 | NLRP3 | NOTCH1 | PARP1 | PCNA | PDCD1 | PLK1 | PRKAA1 | PRKAA2 | PTEN | PTGS2 | PTK2 | RELA | SIRT1 | SLTM | SMAD4 | SOD1 | SQSTM1 | SRC | STAT1 | STAT3 | STAT5A | TAK1 | TERT | TLR4 | TNF | TP53 | TXN | VEGFA | YAP1